Near-Earth Objects, History and Detection
In Part I of this series, we discovered the asteroid Apophis’ discovery and media furor that the initial orbital estimates caused, then described Near Earth Objects (NEOs) and their categories. Apophis was originally thought to have a 2.7% chance of impacting Earth in 2029. After additional observations refined Apophis’ orbit, that chance was reduced to a 0% for 2029, with the potential for an impact in later approaches, depending on its interaction with Earth.
In Part II, I’ll cover:
Early Close Approaches
Astronomers have observed the close approach of comets to the Earth for as long as they have watched the skies. In man’s early history, comets were recognized in myth, religion, and lore as harbingers of fate. The closer and the larger these comets became, the more dire the fate in most cases. Tycho Brahe was the first to attempt to measure the distance of a comet through parallax in 1577 and in 1705, Edmond Halley was the first to successfully predict the return of the comet we now know as Halley’s Comet.

Source: Popular Science Monthly (archive.org)
Comets Approaching within 25 Lunar Distances (pre-1900)
Comet | Designation | Year | Miss (LD) | Note |
Halley | 1P/837 F1 | 837 | 13.0 | |
Comet of 1014 | C/1014 C1 | 1014 | 15.8 | Miss distance Uncertain |
Comet of 1080 | C/1080 P1 | 1080 | 24.9 | Miss distance Uncertain |
Comet of 1132 | C/1132 T1 | 1132 | 17.4 | Miss distance Uncertain |
Comet of 1345 | C/1345 O1 | 1345 | 18.9 | Miss distance Uncertain |
Comet of 1351 | C/1351 W1 | 1351 | 18.6 | Miss distance Uncertain |
Tempel-Tuttle | 55P/1366 U1 | 1366 | 8.9 | |
Comet of 1702 | C/1702 H1 | 1702 | 17 | |
Comet of 1743 | C/1743 C1 | 1743 | 15.2 | |
Lexell | D/1770 L1 | 1770 | 5.9 | |
Biela | 3D/1805 V1 | 1805 | 14.2 |
433 Eros
In 1898, Carl Gustav Witt of the Berlin Observatory discovered the first Near-Earth Asteroid (NEA), now known as 433 Eros, which is also the second-larges NEO we’ve found. Measurement of Eros’ orbit enabled astronomers to measure the distance from the Sun to the Earth. NEAR Shoemaker probe visited Eros with a 1998 flyby and establishing orbit in 2000. Eros’ orbit is well outside Earths orbit and crosses Mars’ orbit, so it poses no risk to Earth.

Source: JPL/NASA
The Modern Era (1900-Present)
69230 Hermes
In 1937, 69230 Hermes was the next NEO to be discovered at just twice the Earth-Moon distance. The telescopes of the time were limited, so astronomers could only track it for just four days. They were unable to determine Hermes’ orbit and were alarmed at the potential to impact Earth or the Moon.
It was only in 2003 that Hermes was reacquired and astronomers determined its orbit accurately. It is now known that Hermes has a highly eccentric orbit that crosses Mars, Earth, and Venus orbits, which makes it difficult to predict its orbit as it interacts with all three planets, but it will not cross Earth’s orbit for the next century.
While retracing the orbit to link up to the 1937 close approach, astronomers determined that Hermes had an even closer approach at 1.7 lunar distances in 1942, but that was missed due to World War II. This was the closest known approach until 1989.
1556 Icarus
In 1968, 1556 Icarus approached within 16 lunar distances. It was the first minor planet to be observed using radar. Both the Haystack Observatory and Goldstone Observatory observed the pass. While the raw spectrum doesn’t mean much to the naked eye, the processed delay-Doppler image shows rough contours:

Source: JPL/NASA

Comet Shoemaker-Levy 9
In 1994, Comet Shoemaker-Levy 9 (CSL9) stunned astronomers and the public with a demonstration of the tremendous damage a comet fragment could do to a planet. CSL9 did more to raise public and political awareness of the dangers of a space impact than any other event. The world was held in awe of the power of the impacts and suddenly we all felt vulnerable.
Carolyn and Gene Shoemaker and David Levy discovered the comet in March of 1993 through the 0.4 meter Schmidt telescope at Mt. Palomar. CSL9 was in a 2-year orbit around Jupiter and the planet’s heavy gravity had torn the comet into 20 pieces in a close pass in July 1992.
NASA’s Galileo orbiter was traveling to Jupiter and was in a position to observe the 1994 collisions from a better angle than Earth-bound astronomers. The fragments were names A through W and impacted Jupiter from July 16 to July 22. Many observatories watched the impacts from Earth, including the Hubble Space Telescope.

Source: GFSC/NASA
Each fragment hit a fresh piece of Jupiter, so astronomers had the chance to see how objects of different sizes caused differing amounts of damage. The fragments threw plumes of debris 1,200 to 1,900 miles high and heated the the atmosphere to 53,000 to 71,000 degrees Fahrenheit. Some of the marks left in Jupiter’s clouds were larger than the Earth! If this comet had hit Earth, we would have faced at least a “nuclear winter” that would disrupt crops and potentially devastate life on Earth.

Source: GSFC/NASA

Source: GSFC/NASA

Source: GSFC/NASA

Source: GSFC/NASA
Deep Impact & Armageddon
Four years later, in 1998, two movies, Deep Impact and Armageddon presented two fictional stories of impacts that have the potential to end life on Earth. While the science in both is questionable, the movies again raised awareness of the public and politicians that we needed to determine the real danger and develop a plan to protect Earth.
Congressional Hearings
With public pressure, astronomers and politicians became proactive, such as holding subcommittee hearings on “The Threat and The Opportunity Of Asteroids And Other Near-Earth Objects“, held on Space Day in 1998:
…I would also like to express my appreciation to the Chairman for agreeing to hold this hearing. As he knows, the potential threat posed by large, Earth -orbit crossing asteroids and comets is a subject in which I have long been interested.
At the same time, I recognize that for Members who are hearing about this issue for the first time, it is a subject that seems more relevant to Hollywood movies and science fiction novels than to issues that should concern Congress. However, I think that after hearing the scientific evidence, Members are likely to be much less skeptical about the relevance of this issue.
As the witnesses will no doubt recount, the Earth has been repeatedly bombarded by objects from space throughout its geological history. Some of the those objects have been more than a kilometer in diameter, and have impacted the Earth with the force of thousands of hydrogen bombs. In some cases the resulting release of energy has had global consequences.
One of the most widely cited examples is the evidence of the impact of a large asteroid off the Yucatan peninsula some 65 million years ago. The geological and paleontological records strongly suggest that the event may have triggered mass extinctions on a global scale, and may have contributed to the demise of the dinosaurs.
Yet the threat posed by such objects is not something confined to the distant past – our planet continues to be bombarded by objects of varying sizes. And scientists estimate that there are some 2,000 Near Earth Objects whose orbits could put them on a collision course with the Earth at some point in the future.
Of course, the critical question is when such a threat might arise. Unfortunately, the honest answer has to be that we don’t yet know . That is because we have not yet detected all of those large, Earth – orbit crossing asteroids and comets, and so we cannot analyze their future orbital paths.
As a nation, we have begun to correct that situation. Due to the dedication of a number of scientists—some of whom are testifying before us today — the detection of Near Earth Objects has become a serious scientific objective. In that context, I would like to acknowledge and honor the efforts of one who is no longer among us — Dr. Eugene Shoemaker. I considered him both a friend and a thoughtful advisor on this and on numerous other scientific issues, and I miss him.
Yet to say that the nation is beginning to focus on the threat posed by Near Earth Objects is not to say that we are doing enough. This Committee has long tried to call attention to the need for a robust and comprehensive program to detect and catalog all of the large Earth -orbit crossing asteroids and comets. I am encouraged that NASA recognizes the validity of such a mission, and that it is attempting to respond to the Committee’s concerns. However; the resources devoted to the task to date have been inadequate. We can and must do more.
Opening Statement by Hon. George E. Brown, Jr. (D, CA), May 21, 1998
House of Representatives, 105th Congress
Committee on Science
Hearing before the Subcommittee on Space and Aeronautics
4581 Asclephinus
In 1998, Palomar Observatory observed the asteroid 4581 Asclephius nine days after it had passed Earth at just beyond the Moon’s orbit. Since this was nearly a kilometer in size and passed so close to the Earth, this event increased the urgency to determine the risk these unknown objects represented.
Detecting Near-Earth Objects
While some dedicated projects started in the 1960s, the most productive surveys started with PCAS in the 1970s and automated sky surveys dramatically increased the number of objects detects.
At the start of the century, the will of the people, politicians, and scientists in the US, Europe, and Asia combined to act, fund, and develop surveys. We emerged from a few solo efforts of scientists to coordinated, computerized, world-wide sky surveys in multiple bandwidths and follow-up observations in less than a decade. Subsequent discoveries showed whole classes of unknown objects around Earth, causing even more nations to join the search.
Sadly, the media beats up a frenzy about any rock that comes close to the Earth or Moon, leading to fatigue at the warnings. Hopefully, politicians won’t tune out when we really need to act.
Significant Past Surveys of NEOs:
Some astronomers made accidental discoveries of NEOs while searching for other solar system objects, but there were five early dedicated systems that have now gone offline. Their legacy lives on as comets often retain the observatory or astronomer’s name:
- Palomar Planet-Crossing Asteroid Survey (PCAS) – 1973 – Eugene Shoemaker and Eleanor Helin started PCAS with the Palomar Observatory in California. In 25 years, the program discovered 95 NEOs, including 17 comets.
- Lowell Observatory Near-Earth-Object Search (LONEOS) – 1993-2008 – LONEOS is run by Ted Bowell at Lowell Observatory in Flagstaff, Arizona. LONEOS is credited with the discovery of 22,077 asteroids
- Near-Earth Asteroid Tracking (NEAT) – 1995-2007 – NEAT was a NASA/JPL and US Air Force survey operating from the Palomar Observatory in California and two telescopes in Hawaii. NEAT had discovered more than 40,000 asteroids, making it one of the most successful programs.
- Lincoln Near-Earth Asteroid Research (LINEAR) – 1998-2018 – LINEAR is a joint project between the US Air Force, NASA, and MIT at White Sands and was responsible for a majority of asteroid discoveries from 1998 to 2005. The system included two 1-meter telescopes with a 0.5-meter telescope to review the discoveries of the other two. LINEAR discovered more than 140,000 asteroids or 24 percent of all know PHAs.
Current NEO Search Programs
- Spacewatch – 1988 – Spacewatch is a University of Arizona project using four telescopes from 0.9-meter to 4-meter telescopes at Kitt Peak Observatory in Arizona, backed by NASA and funded by Congress. The 1.8-meter telescope is the largest telescope dedicated to the discovery of asteroids and comets and Spacewatch is the longest-running program to detect NEOs. Today, Spacewatch is primarily used to follow-up on discoveries by other automated system, so it is likely the system with the most observations of NEOs. Spacewatch has achieved many firsts in NEO observations and continues to provide valuable information in our search to identify NEOs.
- Catalina Sky Survey (CSS) – 1998 – CSS started as a project of University of Arizona using the 0.7-meter Schmidt telescope on Mt. Bigelow Observatory. In 2004, after significant upgrades, CSS became the most productive NEO survey. From 2004 to 2013, the Siding Spring Survey joined CSS to cover the southern hemisphere. In 2014, a new 1-meter telescope on Mt Lemmon joined the system to follow up on the discoveries of the first telescope. Today, CSS has 2 survey scopes and one telescope dedicated to follow-up. CSS has discovered 47% of all known NEOs.
- Campo Imperatore Near-Earth Object Survey (CINEOS) at the Rome Observatory. Located 130km northeast of Rome, CINEOS concentrates on NEOs that remain primarily inside Earth’s orbit, which is an area not well covered by other surveys. However, since Earth is a major gravitational factor in Aten NEOs’ orbits, these are vital areas to observe. CINEOS also provides rapid astrometric data on high-priority NEOs and to reacquire NEOs at future appearances. The observatory has other missions, so CINEOS runs 10-14 nights a month. Despite the part-time usage, CINEOS has discovered more than 1,500 new objects and measured more than 60,000 asteroid positions.
- EUROpean Near Earth Asteroids Research (EURONEAR) – EURONEAR involves 9 countries, 26 telescopes, and 143 scientists with observatories in La Palma, France, Tenerife, Spain, and Romania. The EURONEAR web site has a list of data mining tools to plan observations, identify NEOs, find future appearances, and much more.
- Optical Ground Station (OGS) – The European Space Agency operates the OGS at Tenerife, Spain. The 1-meter telescope with a cryogenically cooled camera (4k x 4k) scans the night sky for space debris as small as 10cm in geostationary orbit and NEOs to 19th or 20th magnitude. It has discovered 37 minor planets.
- Asteroid Terrestrial-impact Last Alert System (ATLAS) – University of Hawaii & NASA – ATLAS will provide one day’s warning for a 30-kiloton “town killer,” a week for a 5-megaton “city killer,” and three weeks for a 100-megaton “county killer”.’ It consists of two telescopes, 100 miles apart, on Haleakala and Maunaloa. ATLAS scans the whole sky several times every night looking for moving objects and the Scout Hazard Assessment system uses computer programs to use the parallax between the two telescopes to determine the distance to the asteroid. (Close objects will have larger movement in front of the background stars.) The data is also used to find stationary transients like supernovae, stellar outbursts, and gamma ray burst afterglows.
- Panoramic Survey Telescope And Rapid Response System (Pan-STARRS) – Pan-STARRS is based at the Haleakalā Observatories on the island of Maui in Hawaii and is famous for discovering some showcase comets and the interstellar object 1I/2017 U1 ‘Oumuamua. Pan-STARRS has two 1.8 meter Ritchey–Chrétien telescopes systems with huge CCD cameras. The individual chips are 4.8k x 4.8k pixels wide. Camera 1 has 60 CCDs in an 8×8 array (minus 4 in the corners), giving a total sensor surface of 400 mm x 400 mm – That’s larger than most amateur telescopes! Camera 2 has 64 CCDs in the same array. Pan-STARRS is unique in that it is searching well outside the ecliptic to find objects with high-inclination orbits and has discovered nearly 5,00 NEOs to date.

Source: neo.ifa.Hawaii.edu
- Near-Earth Object WISE (NEOWISE) – 2010-2023 – The space probe Wide-field Infrared Survey Explorer (WISE) performed an infrared all-sky survey from 2009 to 2010, which was when the coolant was depleted as planned. The mission was extended for four months to test detecting NEOs, then the probe was placed in hibernation. In 2013, the probe resumed searching for NEOs. NEOWISE made 640,000 observations of more than 26,000 known objects and discovered 416 new objects. Roughly 25% of those observations were NEOs and 47 were classified as Potentially Hazardous Asteroids (PHAs).

Gray dots are main belt asteroids, Green dots are NEOs, Yellow squares are comets
Source: JPL
New/planned projects:
- NEOSTEL – European Space Agency observatory with a unique “Flyeye” compound telescope with a 45 square degrees field of view with an estimated limiting magnitude 21. It is a compound telescope with a solid primary mirror and an 16-segment secondary mirror. Each of the 16 beams has its own lens and CCD camera and the computer system integrates the 16 images into the wide field. Estimated to start in 2020, but I could not find information newer than 2018.

Source: ESA
- NEO Surveyor – After several failed attempts to get funding, NEO Surveyor was authorized in 2019. It will orbit in the L1 Lagrange point (directly between the Earth and Sun) and seek asteroids with a 50cm telescope with an infrared camera system operating at two different infrared wavelengths. Its five-year mission is to find at least 2/3rds of the NEOs larger than 140 meters. The two infrared wavelengths will allow NEO Surveyor to accurately measure the size of the asteroid, giving key insight to its rotation, orbit, composition, and shape. It’s also unique in that it is looking forward and rearward in Earth’s orbit with a 45 degree field of view, avoiding the Sun’s glare while catching many asteroids Earth-bound telescopes would miss.

Source: neos.arizona.edu
- Legacy Survey of Space and Time (LSST) – The LSST is being built at the Vera C. Rubin Observatory in Chile. This monster telescope has a unique design that gives the 8.4 meter mirror a 3.5 degree field of view! To achieve this unprecedented field of view, the optics are complicated, with three large mirrors and two lenses. The LSST will us 3.2-gigapixel digital camera with a 64 cm (25.2 inches) diameter sensor and a filter switching system. The camera will shoot 15-second exposures every 20 seconds, then shift slightly and image again. That’s a real challenge for a huge telescope, so it’s built short and extremely sturdy to prevent movement. It is expected to take over 200,000 photos per year (1.28 petabytes!), which will be computer analyzed for significant hits. Alerts will be issued within 60 seconds if an object is detected to have moved or changed brightness between two observations. Scientists predict roughly 10 million alerts each night and these alerts are made public immediately.

Source: LSST.org

Defining NEOS
Now that we’ve discovered a near-Earth asteroid, we need to determine its orbit, composition, and size. That’s not easy from a distance, but scientists have well-established computer-aided processes that generate the data fairly quickly once the asteroid is observed several times.
How Do We Establish the Orbit of a NEO?
Once you have three observations of a body moving against the night sky, you can calculate the orbit. The Asteroid Orbit Determination Project (columbia.edu) has a good explanation of the basics, using a 14″ telescope. The resulting image shows the asteroid 2000 PJ5 moving against the background stars near top center of this image:

The more the asteroid moves against the stars (the more time between observations) and the more accurate the observations, the more accurate your results will be. The algorithm used is complex enough to earn its own page on Wikipedia.
The orbital parameters look rather perplexing, but can be understood:
- Semimajor axis (a) = 1/2 the longest distance in a straight line across the ellipse.
- Eccentricity (e) = a measure roundness.
- e = 0; circular orbit
- I don’t know of any body in a truly circular orbit, but some come close.
- 0 < e < 1 = elliptic (oval orbit)
- Nearly all NEOs are in elliptic orbits.
- e = 1; Parabolic escape or capture orbits
- Parabolic orbits are the borderline between open and closed orbits. Bodies on incoming parabolic orbits are in a capture orbit that will change to elliptical. Bodies on an outgoing parabolic orbit are on an escape orbit.
- e >1 = Hyperbolic escape orbit (used by space probes in gravity assist boost)
- Hyperbolic orbits are escape orbits, where the body has enough energy to escape the main body’s gravity. Comets from the Oort cloud or interstellar space can have hyperbolic orbits, but we are likely to see them only once.
- e = 0; circular orbit

Source: Wikipedia

- Inclination (i) = a measure of the flatness of orbit when compared to the rest of the solar system and is measured in degrees above the ecliptic. (see the diagram below)
- Longitude of ascending node (☊ or Ω) = If nothing else, just remember this term shows the direction of the tilt of the orbit vs. a fixed point in space. This term combines a few references that make it a bit confusing:
- The “ascending node” is one of the two points where the orbit crosses the ecliptic (plane of Earth’s orbit).
- The First Point Of Ares (♈︎) in simple terms is a line to the star Alpha Ari in the constellation Ares.
- FPOA is actually the point that the Earth’s equator (tilted 23.5 degrees) meets the ecliptic during the March equinox.
- Putting it together, if you look down on the solar system with Earth’s north pole showing, the longitude is the angle from the reference line of the First Point of Ares going counterclockwise to the place where the object rises above the ecliptic.
- Argument of perihelion (ω) = Closest approach to the Sun (perihelion) or main body (periapsis) like a planet
- True anomaly (ν) = Fixes the orbiting body to a point on the orbit at a specific time, called an epoch. The most common reference is to noon January 1, 2000, Coordinated Universal Time (UTC). However, newly discovered bodies will use the position and time of discovery.
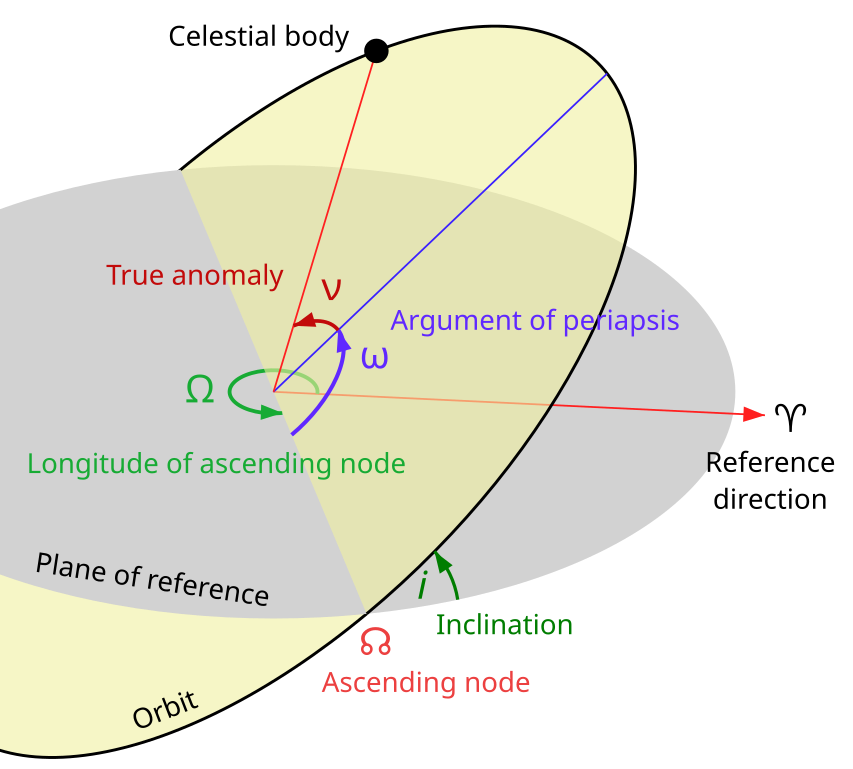
When you put all of these factors together, you have described the orbit and the location of the body in that orbit. You can use these standard orbital factors in astronomical software that will allow the software to calculate a position in the past or future, but it assumes a stable orbit. (Typically, the software will not show what happens when the asteroid comes close to another asteroid, moon, or planet to change the orbit.)
Apophis’ Orbital Elements
Note that most astronomical software amateur astronomers us will to predict gravitational pull of other bodies that change this orbit. Putting Apophis’ orbital parameters into your favorite sky atlas program will show a close encounter at April 13, 2029 21:46 UT, but will not show what happens when it hits the gravitational keyhole.
If you want to tinker, here is the data on Apophis from the Small-Body Database Lookup (nasa.gov):
Epoch 2459396.5 (2021-Jul-01.0) TDB
Reference: JPL 216 (heliocentric IAU76/J2000 ecliptic)
Element | Value | Uncertainty (1-sigma) | Units |
---|---|---|---|
e | 0.1914347568286823 | 1.7124E-9 | |
a | 0.9227004200463054 | 2.5908E-9 | au |
q | 0.7460634895090179 | 1.4143E-9 | au |
i | 3.338874353538208 | 1.104E-7 | deg |
node | 203.9662692226386 | 3.777E-6 | deg |
peri | 126.5900238507279 | 4.1531E-6 | deg |
M | 328.7983451680062 | 9.5747E-7 | deg |
tp | 2459424.558511739019 2021-Jul-29.05851174 | 7.9245E-7 | TDB |
period | 323.7348877947816 0.8863378173710654 | 1.3635E-6 3.7331e-9 | d y |
n | 1.112021019582564 | 4.6836E-9 | deg/d |
Q | 1.099337350583593 | 3.0868E-9 | au |
Note that depending on your program, you may have to convert units or the value notation to make it work.
How Do We Establish The Size Of A NEA?
Once we find an asteroid and fix its orbit, the next step is to stablish size, which helps to quantify the risk it poses to Earth. We can most easily estimate the size based on their albedo (reflectivity). Asteroids of specific types have the same general spectral class (colors) and albedo (reflectivity). These estimates are combined with radar observations when possible for accuracy.
For a thought experiment, imagine a dozen polished tiles in four colors and three sizes mounted on a perfectly non-reflective black board 400 yards from you. Through a telescope, each of the tiles reflects a different amount of light in a different color or spectrum, so they are easy to assign a size based on brightness and color.

Source: Pinterest
While we know a reasonably accurate distance for an asteroid, the problem becomes more complicated when you have little data on the composition of the asteroid. Most asteroids are a tone of gray from weathering by the Solar wind, but they can vary from nearly white to a charcoal color. As you can see in the graphic below, visible light cannot be used to determine the asteroid size.
However, all is not lost, since each asteroid is absorbing heat from the Sun and will have roughly the same heat as any other asteroid. Think of a variety of rocks in an oven or freezer. If left long enough, the small and large rocks will have the same temperature baked into its core, so you could use an infrared (non-contact) thermometer to see they hold the same temperature. Therefore, observing the asteroid in infrared (heat) bands will give us a standard brightness. Since we know a reasonably accurate orbit, we’ll know a good estimate of the distance from Earth and can therefore calculate the absolute magnitude, which is a measure of brightness from a specific distance.

NEOs Discovered So Far
Using these methods, these projects have discovered over 26,000 NEAs and 2,200 PHAs according to CNOES. This represents 90% of the NEOs larger than one kilometer and these projects are now seeking 90% of the NEO population larger than 140 meters. (I’ll cover why 140 meters is important later.)

Source: CNEOS

Sources: Wikipedia and CNEOS
In the next article, I’ll discuss how scientists assign the risk of impact and damage to each NEO and also what to think about all the media frenzy behind the next “big as a sky scraper” asteroid that passes Earth.
Further Reading / Resources
- Interception and Disruption, Johndale Solem, Planetary Defense Workshop, Los Alamos National Lab, 1995
- Meteorites, Impacts, and Mass Extinction, Prof. Stephen A. Nelson, Tulane University, Apr 2018
- Planetary Defense Workshhop Proceedings (archive.org), Lawrence Livermore National Lab, May 1995
- The Threat and Opportunity of Asteroids and Other Near-Earth Objects, House Subcommittee on Space and Aeronautics, May 1998
- In Depth | P/Shoemaker-Levy 9 – NASA Solar System Exploration
- Discovery Statistics (CNEOS)
- National Academies Report on Defending Planet Earth (givewell.org)
More Stories
Sep 17 Lunar Occultation of Saturn
Patroclus-Menoetius Occultation Presentation
Astrophotography Pt. 1 – Equipment