NEO Risk and Mitigation
In Part I of this series, we discovered the asteroid Apophis’ discovery and media furor that the initial orbital estimates caused, then described Near Earth Objects (NEOs) and their categories.
In Part 2, we took a look at NEOs in history and today, plus how we detect and define them.
Part 3 will look at the how we determine and communicate the risk of NEO impact and how we might mitigate them.
- Many NEOs
- General Risk
- How Do Astronomers Assign Risk of Impact?
- Social and Environmental Harm
- Risk Of A Specific Asteroid Impact
- Mitigation – What Can We Do?
- Further Reading / Resources
Many NEOs
In Part 2, we saw that “there are over 26,000 NEAs and 2,200 PHAs according to CNOES. This represents 90% of the NEOs larger than one kilometer and these projects are now seeking 90% of the NEO population larger than 140 meters.”
That’s a large number, so let’s put that into a visual perspective. First, most of the Near-Earth Asteroids (NEAs) originate from the asteroid belt, after being perturbed out of the nearly circular orbit. Most of these NEAs are in elliptical orbits that cross Earth’s orbit, but spend most of their time well inside or outside Earth’s orbit. If they come close to Earth, Earth’s gravity will alter their orbits, sometimes dramatically. This leaves a bit of a chaotic picture:

(Slightly misleading as the space between objects is compressed and the dots are actually larger than the Sun.)
Gold orbit is Mercury. Purple orbit is Venus. White is Earth. Brown is Mars. Gold is Jupiter. The wide band of dots is the asteroid belt.
Source: JPL/NASA
With all of these rocks crossing Earth’s orbit, at least a few represent a real risk of impact. So scientists must first examine the orbits of each to predict if the asteroid approaches or could possibly impact Earth. In many cases, newly discovered asteroids need additional observations to better define the orbit.
For example, Apophis was originally thought to have a 2.7% chance of impacting Earth in 2029. After additional observations refined Apophis’ orbit, that chance was reduced to a 0% for 2029, with the potential for an impact in later approaches, depending on its interaction with Earth.
General Risk of Asteroid Impact
So now we know many objects come close to Earth, how do we determine the risk each object presents to Earth, society, and technology?
According to the latest scientific papers, meteors of various sizes have different average time between impacts on Earth’s atmosphere:
- Daily, the largest impactor will be roughly 16 inches but we will also receive approximately 48.5 tons of meteors of all sizes (dust size and up).
- Objects larger than 2 or 3 cm will partially burn up, but still strike the Earth.
- Annually, the largest impactor will be roughly 9-13 feet.
- Every 100 years, the largest impactor will be roughly 66 feet.
Remember that these general odds have no memory. Even though the 20 meter Chelyabinsk meteor is a fairly rare (approximately 50 years between them), we could see another one any day or not see one for 100 years.
Bolide events are small asteroids that explode from the heat and shock while entering the atmosphere. Government sensors in early warning satellites (monitoring the enemy for missile launches), infrasound ground monitors, American Meteor Society reports and even security cameras monitor the sky for these events. Combining this data leads to a stunning chart showing a large number of impacts over just 19 years:

Size of dot = optical energy (bigger = brighter)
Source: JPL/NASA
But size is only part of the story. We must also consider orbital parameters. Many asteroids that share Earth’s orbit don’t come close to Earth, so no matter the size, they don’t present a hazard to Earth in the foreseeable future. Of the 27,117 Near-Earth Objects, only 2,217 are categorized as Potentially Hazardous Asteroids (PHA). PHA is defined as an asteroid that approaches within 0.05AU or less. Of those 2,217 PHAs, there are no asteroids considered an impact danger to Earth.
Near-Earth orbits can be chaotic, as we saw with Apophis when the close approach dramatically changed its orbit and odds to impact Earth. Asteroids are small bodies that are pulled by the Earth, Sun, other planets, and other asteroids. Each close encounter changes the orbit, which then can influence other asteroids, making predictions of encounters far into the future difficult. Generally, predictions beyond 100 years are more guess than certainty.
The other factors include the speed and angle of impact. An asteroid catching up to Earth or being overtaken by Earth will have far less energy than one on a hyperbolic orbit hitting at a 90-degree angle at high speed. The Chelyabinsk meteor was the weight of an aircraft carrier and had enough speed to generate 320 to 480 kilotons of TNT (More than 30 times the power of the Fat Man bomb.) However, the meteor hit at a very shallow angle, giving it time to heat up and explode at 18.4 miles (29.7 Km) above the surface. While the city saw damage to buildings and people, if that rock had plunged at a steeper angle, it would have obliterated most of the city. The impact angle is hard to determine, since a small change in the actual approach vector changes a near miss to a deep plunge. However, we can predict the approach speed based on the known orbits and plot damage for the worst-case scenario.
How Do Astronomers Assign Risk of Impact?
Once astronomers have observed a new asteroid and enough observations to give an approximate orbit, they must search for impact risk. Since the data is imperfect, the solution will include multiple orbits that fit the observations. The Near-Earth Object Studies (CNEOS) is our subject matter expert and their Sentry system is a computerized search system that uses the margin of error in observations to predict if any of those orbits could possibly intersect Earth.
When interpreting Sentry pages, where information on known potential NEA impacts is posted, one must bear in mind that an Earth collision by a sizable NEA is a very low probability event. Objects normally appear on the Risk Page because their orbits can bring them close to the Earth’s orbit and the limited number of available observations do not yet allow their trajectories to be well-enough defined. In such cases, there may be a wide range of possible future paths that can be fit to the existing observations, sometimes including a few that can intersect the Earth.
Whenever a newly discovered NEA is posted on the Sentry Impact Risk Page, by far the most likely outcome is that the object will eventually be removed as new observations become available, the object’s orbit is improved, and its future motion is more tightly constrained. As a result, several new NEAs each month may be listed on the Sentry Impact Risk page, only to be removed shortly afterwards. This is a normal process, completely expected. The removal of an object from the Impact Risk page does not indicate that the object’s risk was evaluated mistakenly: the risk was real until additional observations showed that it was not. On the other hand, the impact Risk Page lists a number of lost objects that are, for all practical purposes, permanent residents of the Risk Page; their removal may depend upon a serendipitous rediscovery.
Sentry: Earth Impact Monitoring (nasa.gov)
The normal process is for the sky survey systems mentioned in Part 2 of this series work together to reacquire NEOs that were lost in sunlight or distance and to observe newly discovered NEOs later in their orbit. Both types of observations will greatly increase the accuracy of the orbits and reduce the uncertainty of impact risk.
Some of the telescope systems are reserved for this type of work, while most are doing sky surveys that might capture previously lost bodies. Space radar systems are used in cases where high precision is needed for the predictions. Since Apophis was originally thought to have a 2.7% chance of hitting Earth if it passed through an 800m gravitational keyhole approach in 2029, radar precision was needed. As a result, Apophis was downgraded several times until there was a 0% chance of it hitting Earth in the next 100 years.
Social and Environmental Harm of An Asteroid Impact
However, even if we know a specific asteroid has a 100% chance of impacting Earth, we still don’t know the whole story. We must also use the estimated size of the asteroid to predict the effects it would have on Earth:
- The smallest items, up to the size of your fist burn up in the atmosphere to create beautiful meteors.
- A bit larger and the rocks can reach the ground without noticeable damage.
- Larger asteroids like Tunguska and Chelyabinsk were air bursts that damage large areas of the ground without making a crater.
- Beyond that, the atmosphere no longer protects the ground and as size goes up, larger areas are devastated:
- city-sized areas,
- larger regions of the Earth
- Civilization and life-ending impacts
To Put The Danger Of Apophis In Scale:
Meteor Crater in Arizona was created by an asteroid just 50 meters (160 feet) across, resulting in 3-10 megaton explosion creating a 1,200 meter (0.7 mile) crater:
“Calculations show that a meteorite with a diameter of 30 m, weighing about 300,000 tons, traveling at a velocity of 15 km/sec (33,500 miles/hour) would release energy equivalent to about 20 million tons of TNT. Such a meteorite struck at Meteor Crater, Arizona (the Barringer Crater) about 49,000 years ago leaving a crater 1200 m in diameter and 200 m deep.”
Meteorites, Impacts, and Mass Extinction, Prof. Stephen A. Nelson, Tulane University, April 2018

Source: Wikipedia
The Tunguska event in 1908 was created by a body approximately 60 meter (200 feet) in diameter and resulted in a 12 megaton explosion. This explosion was an air-burst of a stony meteor that devastated 2,150 square kilometers (830 square miles) of the Siberian forest, killing an estimated 80 million trees and possibly 3 people. If this had hit a major metropolitan area instead of an isolated forest, the city would be devastated and millions would have died.

Source: Wikipedia
The Chelyabinsk meteor was a famous 20 meter (66 feet) meteor that rocked the city with a huge shockwave as the meteor burst overhead in February 2013. The light from the meteor was brighter than the Sun and was recorded by many dash and security cameras. The blast was roughly equivalent to 500 kilotons of TNT or 30 times the power of the Hiroshima bomb. The shockwave followed the flash of the explosion by several minutes, giving people enough time to go to a window or exit to the street to see what happened. When the shockwave arrived and shattered most windows, flying glass injured 1,500 people.

Source: Wikipedia
Radar and optical observations of Apophis set its size 450m x 170m, which nearly ten times larger than the examples above. That means the energy of an Apophis impact would be one hundred times larger:
- Apophis = 1,200 megatons
- Tunguska = 12 megatons
- Meteor crater = 3-10 megatons
- Chelyabinsk = 500 kilotons
- Little Boy Hiroshima bomb = 15 kilotons
An explosion of this size would create regional devastation and if it hit a highly populated area like the eastern coast of the USA somewhere around Washington DC, many millions would die and many cities would vanish. A water impact in the Pacific or Atlantic oceans would create huge tsunamis that would devastate coastlines on both shores.
Large Asteroid Impacts
The three largest known asteroid impacts were global catastrophes, reshaping the climate and shrouding the Earth in a long, sunless winter.
The Vredefort crater in South Africa is the largest known impact crater on Earth and at 2 billion years old, one of the oldest known. The 10-15 km-wide asteroid created a crater approximately 300 km wide, though much of the crater has eroded away, partial rings of the crater are visible from space.
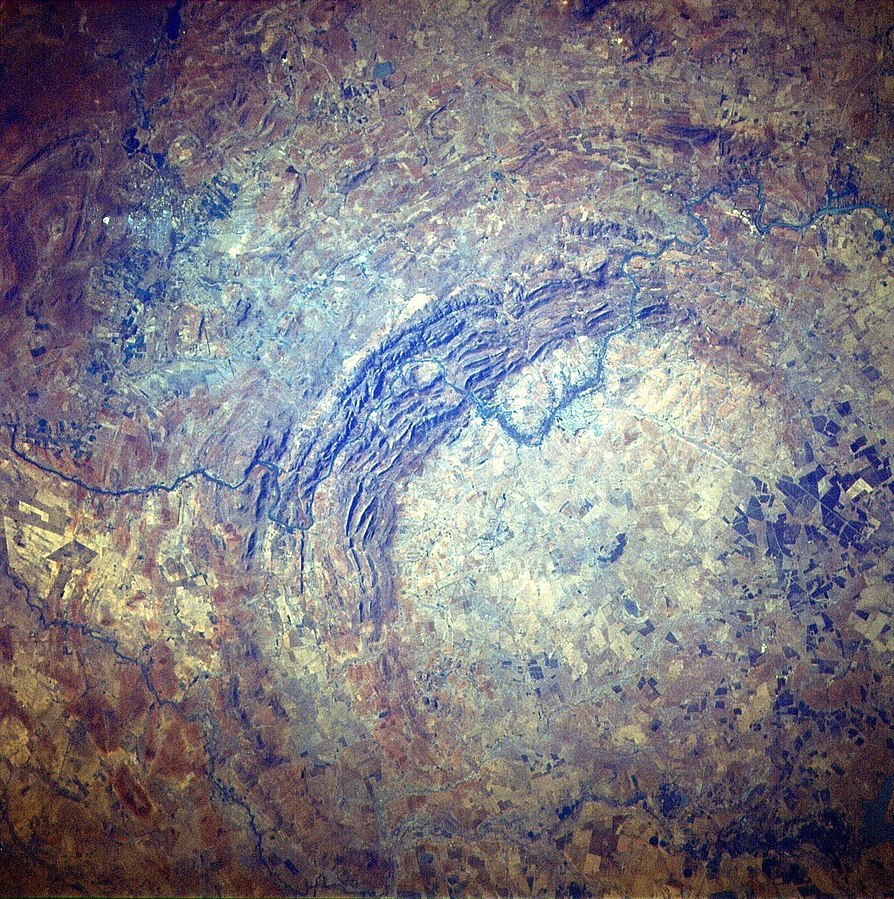
One of the best known impacts was the Chicxulub crater, that was formed when an asteroid of approximately 10 kilometers in size impacted the shore of what is now the Yucatan peninsula. The impact energy has been estimated to be 21-900 billion Hiroshima (15 kilotons) A-bombs. The crater is an estimated 150 kilometers (93 miles) wide and is the second largest impact basin on Earth. The impact caused the Cretaceous–Paleogene extinction event, which caused a mass extinction event of the dinosaurs across the globe.
- The Chicxulub event would have created a tsunami 100 meters tall in the shallow water of the Gulf of Mexico. In deeper waters, the same event would have raised a tsunami 4.6 km tall.
- The debris thrown into space would have heated on reentry, superheating the atmosphere and upon landing, caused wildfires across the globe.
- Dust covered the entire surface of the Earth for up to a decade, making life difficult for the few remaining animals. The dust would also remain in the upper atmosphere for up to a decade, disrupting plant growth.
- The shockwave from the impact would have caused global earthquakes and volcanic eruptions.
- The debris and dust formed a layer that is now preserved in the geologic record that also shows an abrupt end of fossils in this dark layer around the globe.

The Sudbury Basin is 130 km across and is the third-largest known impact crater on Earth. The asteroid punched deeply into the Earth and the resulting magma flow is the source of rich nickel, copper, gold, and platinum ores. The 10-15 km asteroid impacted 1.8 billion years ago and debris was thrown more than 800 km from the impact site.

Risk Of A Specific Asteroid Impact
Now that we’ve looked at NEOs and know they commonly approach Earth, how do we communicate the risk of a specific object poses to Earth, especially when orbital and physical data is not well-known?
- In 1995, Professor Richard P. Binzel from MIT presented the first version of the scale to the United Nations conference and later published in the Annals of the New York Academy of Sciences, volume 822, 1997.
- In June of 1999, a revised version of his scale was presented to the international conference on NEOs held in Torino, Italy and was adopted as the Torino Scale.
- In 2005, thanks to the media “crying wolf” on level 1 asteroids, scientists slightly changed the wording at all levels, including Level 1 that was changed from “Events meriting careful monitoring” to “normal”, which would indicate a normal discovery and would be harder to hype using this scale. Unfortunately, media continue to hype close approaches that pose no real danger.
Torino Scale
How do we communicate it? Setting off the alarm every time a rock comes near, but won’t hit Earth will soon seem like crying wolf. (I’ll stick with scientific and political ramifications and leave media reactions out of this discussion as they cry wolf any time a small rock gets within a lunar distance.)

The Torino scale combines:
- The probability of impact based on all data collected so far
- The potential local, regional, or global devastation (related to size of the object)
- The expected time period for the impact (how much time we have to act)
The scale very quickly shows the danger each asteroid presents to Earth, society, technology, and climate in a very clear way. As we saw with Apophis, the orbits can change, so the Torino rating and odds of impact can change as well.
- White – No Hazard:
- A large NEO has no risk of impact
- A small body that will burn up in the atmosphere.
- Green – Normal (No cause for concern):
- A new discovery with enough data to determine a rough orbit with almost no chance to hit
- Still warrants further observation to be sure
- Very likely rate white with further observations
- Yellow – Meriting Attention (Unknown danger):
- 1% or larger chance of hitting Earth based on early observations.
- Public announcements if less than 10 years from impact
- Further observations needed to refine the orbit and the odds of hitting Earth
- Think of Apophis in the early days of discovery
- Still likely to be downgraded with further observation
- Orange – Threatening (Known danger):
- Object posing serious, but still uncertain threat (orbit still rough but more than yellow)
- Critical attention by astronomers to refine the orbit
- Governments may need to plan contingencies and mitigation.
- Red – Certain Collision (Act now):
- We are in danger, collision is certain.
- Causing localized, regional, or global devastation.
- If there is time, governments will need to plan contingencies and mitigation.
Current Risk
There are no asteroids rated higher than zero/white at this time. Apophis was originally rated level 4/yellow until the orbit was better understood. This was the highest rating of any observed asteroid to date. Many asteroids have been rated 1-3 and later downgraded.
With all this said, you can relax, since the odds of dying from an asteroid impact are far lower than the odds of dying from more Earthly causes, like murder, tornado, flood, or drowning:
Odds of Dying in the U.S. from Selected Causes in a Human Lifetime
Cause of Death | Odds of Death |
---|---|
Motor Vehicle Accident | 1 in 90 |
Murder | 1 in 185 |
Fire | 1 in 250 |
Drowning | 1 in 9,000 |
Flood | 1 in 27,000 |
Tornado | 1 in 60,000 |
Global impact by asteroid or comet | 1 in 75,0000 |
Earthquake | 1 in 130,000 |
Lightning | 1 in 135,000 |
Regional impact by asteroid or comet | 1 in 1,600,000 |
Shark Attack | 1 in 8,000,000 |
Odds of winning Powerball | 1 in 195,249,054 |
http://www.boulder.swri.edu/clark/binhaz07.ppt
Mitigation – What Can We Do?
Now that we have a rough idea of the risk, how can we prevent the impact? Thanks to astronomers’ dedication, we now know the orbits and risk of 90% of the NEOs over 1km and are now seeking 90% of the NEOs over 140m. It is hoped that we can spot the asteroids well ahead of the impact and have a chance to push or pull the asteroid a slight bit so Earth can change its momentum to a safer orbit.
So how do we push asteroids? Don’t forget that these bodies often have a fairly fast spin, can be loose piles or rubble, an icy body, a solid rock, or iron. Our mitigation plans must take all those compositions in mind.
There are many suggested plans to deflect an incoming NEO. The longer we have to work on the object, the smaller the push we can use. Imagine a large rock on an ice rink. If you had to clear the ice for the hockey game in a few minutes, you’ll need a rope and a large truck to pull it away. If you had a day to move it, you might move the rock with person pushing gently. In space, that gentle push could be beamed energy or a single push from a single impactor. The large force might likely be a nuclear surface burst that turns debris into a reactionary rocket fuel.
Given sufficient warning we might try to avert a collision with a comet or asteroid by using beamed energy
Interception and Disruption, Johndale Solem, Planetary Defense Workshop at Los Alamos National Laboratory, May 1995
or by using the kinetic energy of an interceptor rocket. If motivated by the opportunity to convert the object
into a space asset, perhaps a microgravity mine for construction materials or spacecraft fuels, we might try
a rendezvous to implant a propulsion system of some sort. But the most cost-effective means of disruption
(deflection or pulverization) is a nuclear explosive…
Generalizations indicate: (1) asteroids more than 3 km in diameter can be most efficiently deflected with a surface burst; (2) asteroids as small as 3 km can be effectively deflected with a stand-off device; (3) smaller asteroids are best pulverized….
We would like to find solutions other than nuclear explosives. Clearly, the arms-control, safety, and nonproliferation implications are horrendous. But a practical technology beyond nuclear explosives has yet to emerge. The most nearly competitive technology is the kinetic energy device. The specific energy of an interceptor spacecraft at typical orbital speeds is several hundred times that of high explosive. However, the specific energy of a nuclear explosive is several million times that of high explosive. The kinetic energy device to deflect a kilometer-size object is an unimaginable leviathan (Solem, 1993a; Solem, 1993~). At this time, and probably for decades to come, the only thing we have is a nuclear explosive.
He continues in the paper to describe several modes of engagement:
- Surface-burst device – An explosion on the surface turns surface material into reaction mass, transferring momentum into the asteroid. However, this would create a crater and possibly split the asteroid without giving it the desired change in direction. (A solution to this, not mentioned in Solem’s paper is to use energy weapons like lasers or particle accelerators to more slowly induce the same type of reaction mass with far less danger of fracture.)
- Stand-off device – An explosion off the surface that would throw x-rays, gamma rays, neutrons, and some debris from the device itself to create a reaction mass from a larger area, reducing the risk of fracture. However, because the stand-off device would create far less reaction mass than a surface burst, the device would need to be at least 40 times larger.
- Penetrator device – A bunker-buster type device would bury into the asteroid and fracture the asteroid. There are many problems with this approach, most related to the need for an exact hit to an exact depth at the exact right time to ensure the pieces go in the desired direction. We also lack deep understanding of how the fragments would gravitationally interact, potentially collecting back into an object nearly the size of the original. Surface-burst and stand-off approaches are far less sensitive to those variables.
Fortunately, nukes in space are not the only options, if we have an extended time to work on the asteroid:
Projects in Planetary Defense
- NEO Deflection App: The Jet Propulsion Laboratory has developed a web app that helps examine how a large impact mass could deflect an asteroid at key moments of its orbit. It also shows when you would need to launch a vehicle to catch that asteroid and the sizes that could be deflected with a single space vehicle. The page offers hypothetical asteroids to test cases.
- Asteroid Capture: A small space vehicle to capture and redirect a 7-10 meter, roughly 500 ton NEA to a stable orbit between Earth and the Moon for later manned missions to the asteroid. The project was cancelled, but shows how the vehicle might move smaller asteroids from a collision course.
Further Reading / Resources
- Interception and Disruption, Johndale Solem, Planetary Defense Workshop, Los Alamos National Lab, 1995
- Meteorites, Impacts, and Mass Extinction, Prof. Stephen A. Nelson, Tulane University, Apr 2018
- Planetary Defense Workshhop Proceedings (archive.org), Lawrence Livermore National Lab, May 1995
- The Threat and Opportunity of Asteroids and Other Near-Earth Objects, House Subcommittee on Space and Aeronautics, May 1998
More Stories
Presentation – Messier Marathon & Space News
Feb 7, 2025 – Join us for First Friday Art Trail
Feb 1 Ransom Canyon Star Party Canceled